Based on the recently proposed Liquid/Supercritical CO₂ Hypothesis, a research team including ELSI’s graduate student Shotaro Tagawa and Associate Professor Kosuke Fujishima successfully simulated a subsurface supercritical CO₂–water two-phase environment using a high-pressure reactor. Their study demonstrated the functional significance of this environment in the origin of life. In this setting, water dissolves into the supercritical CO₂ phase, enabling concentration of dissolved ions and organic molecules through dehydration. Additionally, water coexisting with the CO₂ fluid becomes acidic, facilitating the dissolution of phosphate from phosphate minerals (such as apatite). The researchers confirmed that under such conditions, phosphorylation of nucleoside precursors proceeds at temperatures above 60°C. This study experimentally validates that the coexistence of supercritical CO₂ and water on early Earth could overcome two major challenges—the hydrolysis problem and phosphate depletion—providing a stable source of phosphate-containing organic compounds crucial for the origin of life.
In the context of the early Earth, warm and dry terrestrial hot springs have been considered favourable environments for concentrating simple molecules and promoting reactions that lead to the formation of biomolecules, such as nucleic acids and proteins, utilised by life. Conversely, environments rich in water, like modern hydrothermal vents, have been thought to inhibit concentration of organic and inorganic components as well as promoting the hydrolysis of macromolecules. This paradox, known as the “Water Paradox,” has long been recognized as a critical challenge for the origin of life.
In 2022, Takezo Shibuya and Ken Takai from the Japan Agency for Marine-Earth Science and Technology (JAMSTEC) proposed the Liquid/Supercritical CO₂ Hypothesis as a potential solution to this challenge [1]. This hypothesis suggests that CO₂ fluids, which are hydrophobic, could act as a drying agent in subsurface environments, similar to the role of the atmosphere in terrestrial settings. Particularly, supercritical CO₂, which exists above 31.1°C, exhibits unique properties of both gases and liquids, creating a highly hydrophobic environment even in water-rich subsurface conditions. Furthermore, the acidic nature of water enriched with CO₂ could facilitate the dissolution of phosphate from minerals like apatite, potentially addressing the issue of phosphate scarcity.
To investigate these possibilities, an international research team led by Tagawa and Fujishima examined whether a supercritical CO₂–water two-phase environment could facilitate the dehydration and concentration of organic compounds and release essential ions through mineral dissolution. The supercritical CO₂–water environment was reproduced inside a hydrothermal reactor system and a series of experiments were conducted to validate phosphorylation of nucleosides and the dissolution of phosphate minerals [2].
For nucleoside phosphorylation, initially sodium phosphate salt was used as a phosphate source, and urea was added to promote the reaction. Phosphorylation of RNA precursors—uridine, adenosine, cytidine, and guanosine—were confirmed under the supercritical CO₂–water conditions. Interestingly, cyclic nucleotides, which are commonly observed in wet-dry experiments under conditions simulating terrestrial hot springs, were not detected. Further investigations on uridine revealed that phosphorylation did not occur in water-only conditions without the presence of the supercritical CO₂ phase, highlighting the importance of the two-phase environment. Additionally, the yield of phosphorylated uridine increased with rising temperatures (60°C–94°C).
In the apatite dissolution experiments, it was observed that lower temperature conditions (25°C) resulted in higher phosphate concentrations dissolved from apatite. When apatite was used as the phosphate source, the phosphorylation of uridine was confirmed, albeit with lower yields compared to using sodium dihydrogen phosphate. These results suggest that the supercritical CO₂–water two-phase environment enables phosphate mineral dissolution at lower temperatures, supplying the key resource that facilitates organic phosphorylation at higher temperatures. Observations using a reactor with a glass window also revealed water evaporating into the supercritical CO₂ phase, indicating that this environment could provide conditions analogous to terrestrial hot spring sites, where molecules are concentrated through dry-up.
This study experimentally validates a key functional role of abundant subsurface CO₂ environments in prebiotic chemical evolution. It also provides new insights into resolving the long-standing Water Paradox associated with hydrothermal vent theories, thereby advancing our understanding of the origins of life.
[1] Shibuya, T., and Takai, K. (2022). Progress in Earth and Planetary Science, 9(1), 60.
[2] Tagawa, S., et al. (2024). Astrobiology, 24(12), 60. 1151-1165
Liquid chromatography analysis of products from the phosphorylation of uridine under various temperature conditions in a supercritical CO₂–water environment. The results show that as the temperature increases, the yields of various nucleotide products (UDP*, 5’-UMP, 3’-UMP, 2’-UMP*) also increase. Compounds marked with an asterisk (*) were identified based on mass spectrometry due to the lack of direct comparison with standard substances.
Reproduced from Tagawa et al., Astrobiology 2024.
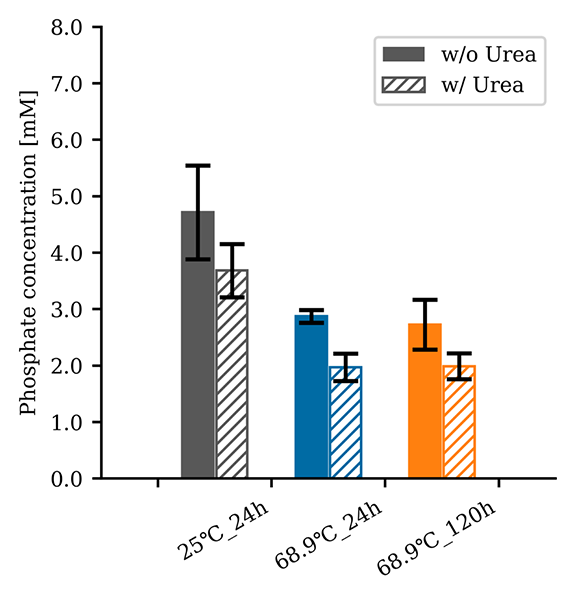
This graph represents the concentration of phosphate released from the phosphate mineral hydroxyapatite into water under a supercritical CO₂–water environment, measured using a phosphate assay kit. Experiments were conducted with and without urea under varying temperature conditions. Error bars indicate the standard error derived from a sample size of n = 3.
Reproduced from Tagawa et al., Astrobiology 2024.
Journal | Astrobiology |
Title of the paper | Prebiotic Nucleoside Phosphorylation in a Simulated Deep-Sea Supercritical Carbon Dioxide–Water Two-Phase Environment |
Authors | Shotaro Tagawa1,2,7, Ryota Hatami3,4, Kohei Morino1,2, Shohei Terazawa1,2, Caner Akil5, Krisitin Johnson-Finn6, Takazo Shibuya7, Kosuke Fujishima1,2,8* |
Affiliations |
|
DOI | 10.1089/ast.2024.0016 |
Online published date | 18 November2024 |