Researchers experimentally demonstrated that hydrothermal vent precipitates, located deep in the ocean, contains aligned nanopores that enable selective ion transport, thus facilitating osmotic energy conversion from ion gradients. A key finding is that selective ion transport, typically associated with biological cell membranes, can occur through naturally formed inorganic nanostructures in geochemical settings. This discovery offers new insights into how life-sustaining energy harvesting processes can arise abiotically from long-lasting chemical disequilibria in deep-sea hydrothermal vents. Additionally, it highlights potential strategies for developing blue energy technologies utilising salinity gradients.
In the deep sea, where sunlight cannot reach, massive structures known as hydrothermal vents (HVs) rise from the ocean floor. These chimney-like structures continuously release hot water containing various metal ions into the cold seawater, gradually growing over time, sometimes reaching heights of up to 60 meters. These vents also support a unique ecosystem distinct from that on the Earth’s surface.
In recent years, similar structures have been discovered not only on Earth but also on ice-covered celestial bodies like Saturn’s moon Enceladus. Since HVs may exist on ancient Earth before life emerged, researchers believe they may have played a crucial role as “natural chemical synthesis systems,” potentially contributing to the origin of life on Earth.
An international team led by researchers from the Earth-Life Science Institute (ELSI) and RIKEN Center for Sustainable Resource Science studied HV samples collected from the “Shinkai Seep Field,” located on the northwestern slope of the Mariana Trench, one of the deepest trenches on Earth, at a depth of approximately 5,700 meters. Alkaline hot water generated from the reaction between olivine and water forms white smoker-type HVs primarily composed of a mineral called brucite, which has a plate-like structure (Figure 2a).
Microscopic observations of the collected samples revealed that small plate-like crystals, approximately 100 nanometers in size, assembled to form a thick membrane, creating a pathway for hot vent water and seawater (Figure 2b). These membranes exhibited periodic stripe patterns, originating from the stacked multiple layers, and grew to a thickness of 200 to 400 micrometers (Figures 2c, d, e).
The researchers conducted synchrotron X-ray diffraction experiments to investigate the structure of the mineral membranes in detail. Multiple regions of the sample were scanned with X-rays, and the directions with the strongest diffraction intensity were indicated with arrows (Figure 3a). In this figure, the alignment direction of the brucite crystals is colour-coded. Remarkably, throughout the scanned sample, the plate-like brucite nanocrystals were found to be orderly and continuously arranged, radiating outward from the vent fluid channel to the seawater (Figure 3b). This arrangement confirmed that nanopore structures suitable for ion transport were formed throughout the entire sample, which has a height of 80 cm.
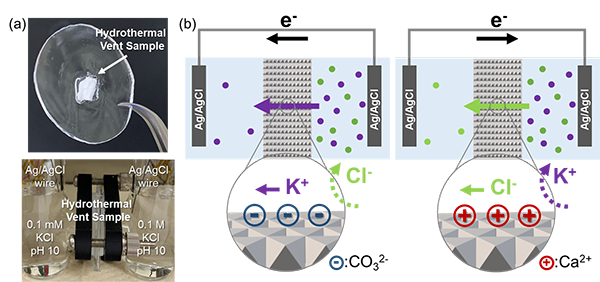
All living beings generate energy by leveraging ion concentration differences within their cells. Therefore, the question of how life began to utilise this mechanism is a crucial in the origins of life. The results of this study demonstrate that energy conversion utilizing ions essential for life can occur naturally through geological processes. Concentration differences of ions are widely observed in nature, and similar phenomena may have taken place on ancient Earth before the emergence of life. Additionally, recent research has confirmed hydrothermal activity on ice-covered celestial bodies. In the future, samples from these celestial bodies may be brought back to Earth for detailed analysis, potentially revealing similar structures and energy generation phenomena.
Journal | Nature Communications |
Title of the paper | Osmotic energy conversion in serpentinite-hosted deep-sea hydrothermal vents |
Authors | Hye-Eun Lee1,2, Tomoyo Okumura3, Hideshi Ooka1, Kiyohiro Adachi4, Takaaki Hikima5, Kunio Hirata5, Yoshiaki Kawano5, Hiroaki Matsuura5, Masaki Yamamoto5, Masahiro Yamamoto6, Akira Yamaguchi1,7, Ji-Eun Lee1, Hiroya Takahashi1,2, Ki Tae Nam8, Yasuhiko Ohara9,10,11, Daisuke Hashizume4, Shawn Erin McGlynn1,2, Ryuhei Nakamura1,2 |
Affiliations |
|
DOI | https://doi.org/10.1038/s41467-024-52332-3 |
Online published date | 25 September 2024 |